1. Introduction
Ice hockey is a team sport that consists of multiple technical tasks such as skating, sliding, shooting and body checking and is organized with phases of play interspersed with passive recoveries (1). As an intermittent team sport, it requires attributes of acceleration, speed, power, endurance and the ability to repeat short and intense efforts (2). The physiological demands of modern ice hockey have increased over the last decades (1, 3, 4). Performance assessment has become a field of expertise that is crucial for researchers and practitioners (e.g., coaches, strength and conditioning coaches, scouts, program directors), who need to be aware of the mechanisms that predispose hockey players to perform in key situations (5). In this regard, several areas of interest are being studied: talent identification, team selection processes, performance analysis, and monitoring individual progress after strength and conditioning training (6). Conducting testing protocols are useful to determine potential and capacity to perform as well as readiness for competition in ice hockey (7). By assessing athletes and allowing a better understanding of determinants of the performance, the process of optimizing these physical attributes becomes part of player development (2, 8). As an example, it has been shown that athletes’ on-ice performances can be improved by developing their athletic capacities with strength and conditioning (9, 10). However, further research is still needed to provide clear scientific evidence that supports the associations between functional fitness, on-ice testing protocols and game performance in real settings (11).
1.1. Testing in ice hockey: from strength and conditioning room to real-game settings
Performance in ice hockey is assessed from three main scientific perspectives: off-ice fitness or athletic capacities (12), on-ice specific fitness attributes (13, 14) and on-ice game performance (15, 16). Although previous researchers have studied functional fitness evaluation for both the youth (17, 18) and professional levels (19, 20), this approach is essential for adapting strength and conditioning training programs to an athlete’s needs (2). Off-ice assessment methods commonly used (i.e., jumps, acceleration, speed, change of direction, upper and lower-body strength, shuttle run, balance), are useful for establishing an athlete’s profile in order to monitor their progress over an entire season (7, 21). There is an extensive description of the testing batteries used, and their role in reaching the high levels of athlete development is well defined (22). The most common one is the NHL Draft Combine (23), which consists of a group of multiple off-ice tests. In this regard, they were shown to have weak predictive validity, especially since they may be less specific to the requirements of on-ice demands (24).
To counteract the limitations of off-ice tests, scientific interest has focused on on-ice fitness specifically in recent decades. The first on-ice testing protocols were conducted over twenty years ago with an emphasis on aerobic capacity, acceleration, speed and change of direction (25, 26, 27). On-ice performance can be assessed in multiple ways, from the empirical evaluation of on-ice skating test times with stopwatches or photo-electric cells (18, 28), modern technologies (14, 29, 30) and biomechanical and kinematics pattern movements analysis (31, 32, 33). Finally, diverse approaches allow for in situ assessment, where it becomes possible to measure and track players’ instantaneous on-ice performance during in-game situations. Previously analyzed with traditional methods (e.g., video analysis or qualitative observation grids) (34, 35), technological improvements have promoted the accuracy and reliability of on-ice performance assessment during game situations (e.g., accelerometry, local positioning systems or automated analysis software) (36, 37, 38).
In summary, we can conclude that two performance-assessment approaches coexist in the domain of ice hockey: “off-ice” fitness tests and specific “on-ice” tests. As mentioned previously, given that off-ice tests may not be specific enough to the requirements of ice hockey, increased attention to on-ice assessment seems to offer the path to a better understanding of the mechanisms underlying performance in ice hockey (39). In this regard, systematic reviews focused on longitudinal off-ice fitness, physiological parameters and on-ice evaluation (40) as well as on-ice performance testing with an emphasis on straight sprint acceleration and speed (41). Nevertheless, many challenges remain when researchers attempt to establish associations between attributes tested on the ice and how these attributes translate into real performance in competition settings (42).
1.2. Objectives: bridging the gap between research and application
When seeking the most reliable and valid options for testing hockey players, researchers face multiple options regarding the best ways to assess the attributes needed to excel. Regarding a major specificity of this sport, there is currently no exhaustive literature review, to our knowledge, of on-ice test protocols used with specific populations and different levels of expertise. From this perspective, bridging the gap between science and its application in practical settings (e.g., less controlled environments) is relevant for both researchers and practitioners. For researchers, an in-depth knowledge of the methods used to assess ice-hockey attributes, with regard to strong external validity, is valuable in their search to replicate research designs specific to the populations studied. More practically, a complete repertoire of on-ice testing protocols is relevant for ice hockey and strength and conditioning coaches as well as federations because it allows them to select methods that are appropriate and adapted to their players’ characteristics (e.g., sex, age, level of play, etc.). Accordingly, the aim of this systematic review is threefold: (1) to present an inventory of the on-ice testing protocols most frequently used to assess physiological and physical attributes relating to ice hockey; (2) to provide a critical overview of the characteristics used in this research including population under study (playing level, sex, age group), study design; validation; and outcomes measures, and (3) to propose recommendations for research-practitioners on the methods that were used in their areas of interest. These recommendations will serve as a framework for designing replicable designs (e.g., in research) and/or implementing testing sessions adapted to stakeholders’ purposes (e.g., fitness testing, team selection, assessment goals, required equipment, etc.).
2. Methods
2.1. Identifying the research question
The systematic review process is a suitable and appropriate method to quantify several studies with different designs, establish links between them and synthesize them (43). This type of design allows researchers to answer questions such as “How many on-ice tests are mentioned in scientific research that aim to assess the physical, technical and physiological qualities of hockey players?” Next, it describes the population, type of study design and observed associations of the different tests in order to identify current contributions and related scientific shortcomings in the field of ice hockey. As mentioned earlier, the main objective of this review is to inform researchers of what has been done in on-ice performance testing and provide measurement tools for practitioners to assess hockey players.
2.2. Finding relevant studies
Article identification and selection was done in accordance with PRISMA guidelines (44), as illustrated in Figure 1. An initial search in three main sport science databases (PubMed, SPORTDiscus and Scopus) was performed on August 2022 using the terms ice hockey and test* as keywords for all databases searched. Searches were limited to articles in English published since January 2000. We justify this time frame for many reasons. Firstly, the introduction of professional (NHL) players at the 1998 Nagano Olympics contributed to the globalization of ice hockey, which resulted in an increase of scholars’ interest towards the science of ice hockey. The profile of modern ice hockey players also changes over the years. For example, an article published by Triplett and colleagues (45) showed that National Collegiate Athletic Association hockey players morphology has changed over the last decades. This suggest that the level of athleticism that is needed to excel in ice hockey might be different than it was in the late 1990’s. Some other factors, such as rule changes (e.g., removing the blue lines, introducing 3 vs. 3 overtimes, etc.) and the emergence of multiple junior-prospects tournaments also contributed to make ice hockey evolve in terms of the required attributes to attain the highest standards. A final argument that could be used is that, from the 2000 s to the present, we have seen an acceleration in the rate of ice hockey publications (46), explaining our decision of a “2000 to 2023” time frame.
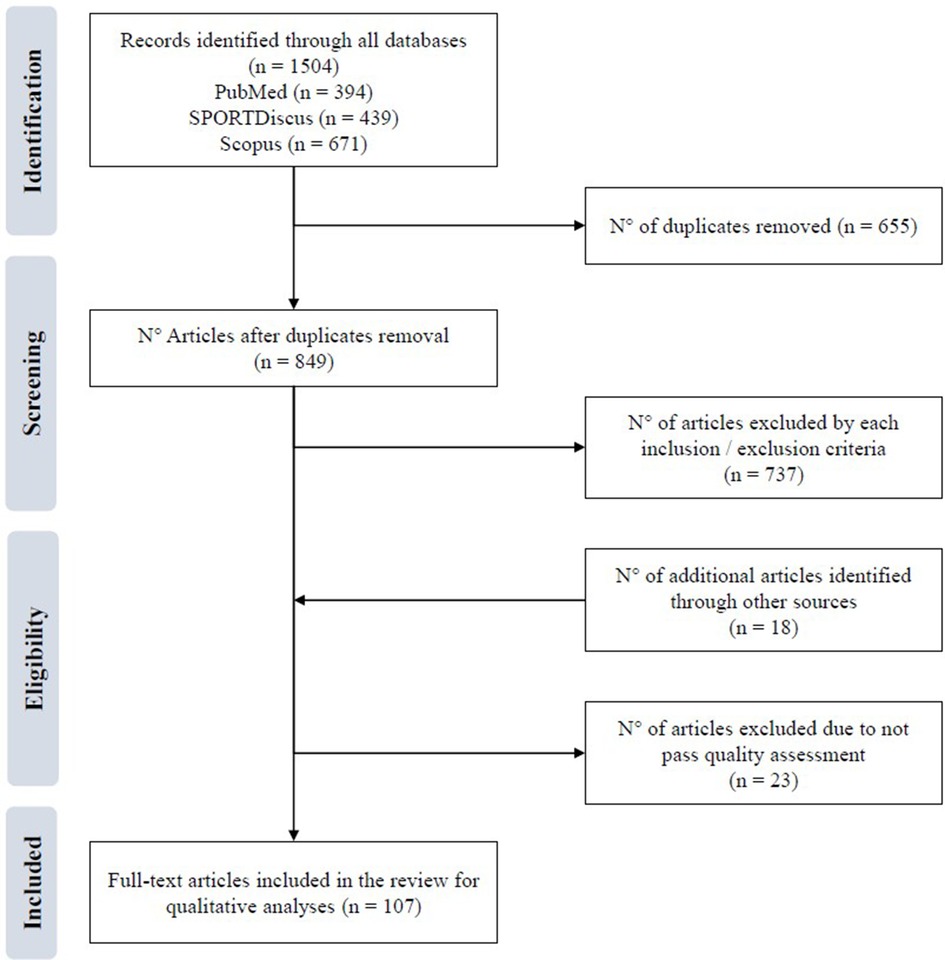
Figure 1. Stages of systematic review-PRISMA to identify on-ice tests in ice hockey.
Since then, speed and agility have become an important aspect of the game. To obtain specific information regarding the validity of the selected tests, we looked for the original study without noting year of publication (between 1950 and 1999). Next, we added articles published from August 2022 to May 2023 as well as articles which were not in the databases mentioned above but which corresponded to the inclusion criteria. Endnote (Clarivate, London, United Kingdom) was used and, after all titles of the three databases were uploaded, the software automatically identified and removed duplicates. Relevant articles were then screened for eligibility after reading the title and abstract of each remaining record, following the same manual PICOS procedure strategy (Population, Intervention, Comparison, Outcomes and Study design) as for the database search and inclusion criteria (47).
2.3. Selecting relevant studies
Two researchers (MB and GM) independently screened the title and abstract for each selected record by applying the PICOS framework. Inclusion criteria were: (1) male and/or female ice hockey players; (2) any articles containing at least one on-ice hockey test; and (3) ice hockey on-ice test that evaluates aerobic capacity, speed, agility or change of direction and ability to repeat sprints. Exclusion criteria for eliminating irrelevant records were: (1) article was not written in English; (2) test was not performed on the ice; (3) testing procedure was not clear (e.g., skating distances or test explanations); and (4) there were no test assessing attributes other than those mentioned above (e.g., technical on-ice test). After completing these steps, the researchers read each eligible article in full to narrow the list to all relevant studies that answered the research question. If the evaluating authors disagreed about the inclusion of an article, the decision was made by a third researcher (JL), also after reading.
2.4. Classification of information
Based on ice hockey game performance analysis (1, 4, 48), we identified four categories of physiological and physical attributes associated with the sport requirements: (1) aerobic capacity, (2) skating acceleration and speed, (3) change of direction (CoD) and agility, and (4) Ability to repeat sprints. Aerobic capacity was defined based on physiological considerations (3, 48) and refers to the use of an incremental protocol where objective measures (e.g., skating speed, distance) increase are observed throughout the test, while subjective parameters are following the same path (e.g., heart rate, intensity, blood lactate) until exhaustion. In speed assessment, a short maximum effort is exerted once in a linear or circular fashion (28, 41). Agility tests refer to efficiency in executing preplanned changes of direction such as tight turns, braking, crossovers and transitions from one skating technique to another over a short distance (26, 49, 50). As shown by Novak and colleagues (50), the transfer from off-ice agility to on-ice skating agility seems plausible in terms of trainability among cohorts of elite under16 (U16) Czech players. A fourth component, which is repeated skating sprint ability (RSSA) is the capacity to reproduce intense or maximal short duration efforts interspersed with brief recoveries (51). However, this ability to repeat such intense efforts involves both aerobic (e.g., high number of repetitions, 60 s or less brief and partial recovery) and anaerobic (e.g., short duration intense efforts less than 10 s) energy systems at the same time. RSSA is therefore considered an important attribute of the sport in ice hockey (48, 52). Furthermore, we are aware that hockey skills are a key component of the toolbox a hockey player uses to excel during a game. Thus, most ice hockey federations have developed testing batteries to test hockey players’ skills at different stages of their development. The International Ice Hockey Federation (IIHF) tests for talent identification and Hockey Canada’s National Skills Standards and Testing Program are good examples of such materials (53, 54). However, we decided to exclude these kinds of protocols, since the aim of this review is to identify tests used in a research context focused on on-ice physiological and athletic testing. For each category of physical attribute mentioned above, we classified all information based on four aspects: population characteristics, reported on-ice tests, outcome measures and main findings.
2.5. Population
For each study retained, we recorded the population’s age (mean), sex, level of play and geographic location. For the mean age, the standard deviation was not considered for classification into the four age groups, which were categorized based on the Long-Term Athlete Development model (LTAD) (55): (1) under 12 years; (2) 12–15 years (youth); (3) 16–19 years (early expertise); and (4) 20 years and +(advanced expertise). In cases involving more than one age group, each subgroup was considered in the number of studies. Sex was classified as male and female. Three playing levels were categorized: youth hockey, competitive amateur (college, university and junior), and professional level. Studies including more than one playing level were classified for all levels under study. The variable “place” was classified according to geographic context: Europe, North America and other (Asia and Australia).
2.6. Types of associations
Types of associations were analyzed to specify the context in which studies were conducted and were classified based on selected studies’ outcomes measures: physiological variables, talent identification, training effects (e.g., following interventions), validation, test parameters, off-ice testing, and other measures (e.g., biomechanical, impact of equipment/nutrition). The authors noted three categories of research designs: observational, (quasi) experimental and validation studies. Researchers gathered all information by formatting an Excel document to include these details. They then extracted the information by attributing a numeric code to each article to classify and analyze the distribution of each type of study.
3. Results
3.1. Selection of articles
Figure 1 illustrates the PRISMA procedure followed for article selection. A total of 1,504 articles were found through a search of three databases: PubMed (n = 394), SPORTDiscus (nn = 439) and Scopus (n = 671). The combined database search yielded 849 titles after removal of duplicates, and 18 studies were added manually (Figure 1). Analysis of the titles and abstracts of each article resulted in the identification of 148 studies for full text review. Among these, 23 studies were excluded for failure to meet quality assessment criteria. At completion of the qualitative analysis process, 107 studies met all the eligibility criteria and were included in the statistical analyses, resulting in 55 on-ice tests. Table 1 provides a summary of the articles in the literature: a total of 107 articles representing 55 on-ice protocols. As displayed, results indicate that tests were designed for assessing aerobic capacity (n = 7), skating acceleration and speed (n = 6), agility-changes of direction (n = 23) and repeated skate sprint ability (n = 19).
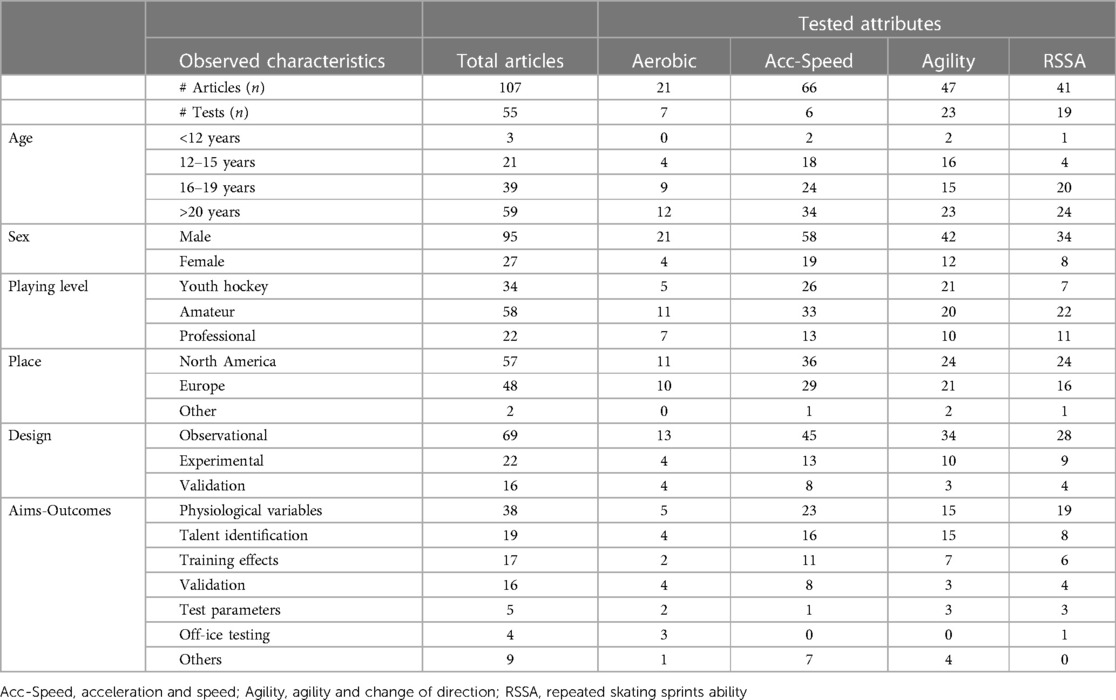
Table 1. Summary of articles reviewed.
3.2. Descriptive results
Table 1 examines a general overview of the included articles, with emphasis on each tested attribute and study parameters (i.e., age, sex, level of play, location, type of associations).
3.2.1. Population characteristics
3.2.1.1. Age
For the populations studied, the age groups most frequently tested in the scientific literature included players over 16 years old, divided into those over 20 years (n = 59) and those 16–19 years (n = 39). Players under 12 years and 12–15 years old were less tested (respectively n = 3; n = 21). Specific to the categories of athletic attributes, older cohorts (e.g., ≥16 years old) were most frequently assessed for on-ice acceleration-speed and ability to repeat skating sprints. Conversely, younger groups of athletes (e.g., <15 years old) were mainly tested on skating agility and on-ice acceleration-speed attributes.
3.2.1.2. Sex and playing level
Regarding sex, males were by far most frequently tested (n = 95) compared to females (n = 27), for each tested attribute. Some differences were observed according to level of play, where results indicate that the literature focused more on amateurs (n = 58) than youth or professional level players (respectively n = 37 and n = 22). On-ice speed tests were mainly administered among amateur cohorts (n = 33), youth assessment focused more on speed and agility components (n = 26; n = 21), and professional athletes were tested in similar proportions on all physical attributes.
3.2.1.3. Geographic location
Results show that geographic location was well distributed. More on-ice evaluations were conducted in North America (n = 57) compared to Europe (n = 49), and only two studies were carried out in other countries (Asia and Australia).
3.2.2. Design
Most of the research done in on-ice hockey testing consisted of observational studies (n = 69), while experimental and validation protocols were less frequent (respectively n = 22 and n = 16). These results are similar when the focus shifts to each specific attribute, with a primary focus on on-ice acceleration and sprinting qualities over other attributes.
3.2.3. Aims and outcomes
Results demonstrate that the main objective of research conducted in ice hockey on-ice assessment relates to physiological variables (n = 38). Below, talent identification, training effect and validation are subsequent outcomes showing similar proportions (ntalent = 19; ntraining = 17; nvalidation = 16). Then, test parameters, off-ice testing and other studies objectives were implemented in the same ratio (respectively n = 5; 4 and 9). On-ice acceleration and speed components along with agility were the two major athletic attributes assessed in the focus on on-ice testing in hockey research.
3.3. Summary of articles included in the systematic review
Table 2 presents results from all the retained articles that focused on on-ice testing in ice hockey. For each article, specifications in regard with population characteristics, reported test, outcome measures and main findings are presented.

Table 2. Basic characteristics of included articles focused on on-ice hockey testing.
3.3.1. Descriptive results with physical attribute focus
Table 3 examines a specific overview of the most used on-ice protocols in the scientific literature for each physical attribute tested. Results present tests that appears three times or more in the literature.
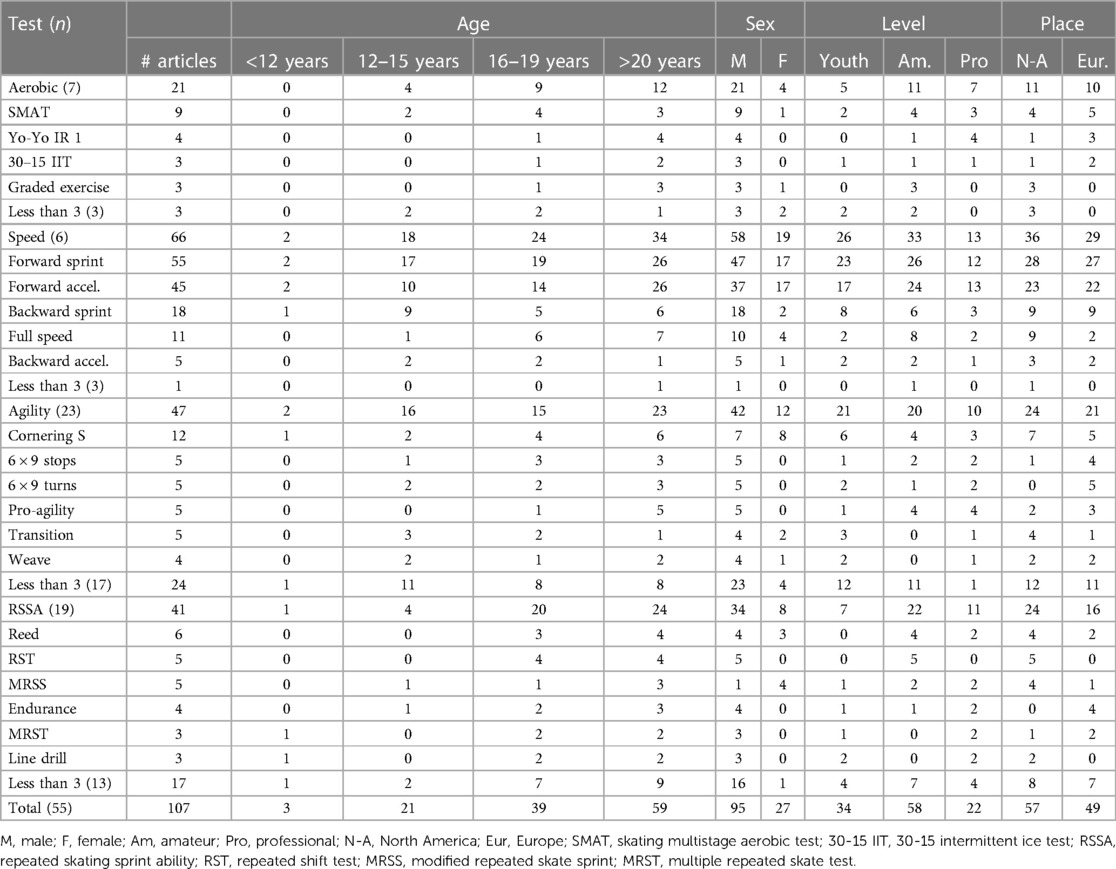
Table 3. Classification of articles by tested attribute with emphasis on the most used on-ice protocols.
3.3.2. Aerobic capacity
A total of 21 articles were found including tests of on-ice aerobic capacity in ice hockey players. The authors listed seven different tests where the majority of studies involved ice hockey players who were 20 years and older (n = 12), were male (n = 21) and played at the amateur level (n = 11). No studies were found on the on-ice aerobic capacity of ice hockey players under 12 years old. Additionally, four studies assessed on-ice aerobic capacity in female ice hockey players. Two of these were designed to test validation, while the others discussed the differences in the physiological parameters of males and females during graded exercise (75). Skating Multistage Aerobic Test (SMAT) is the on-ice aerobic protocol that appears most frequently in articles in the literature, most often regarding the age ranges of 16–19 years (n = 4) and 20 years+ (n = 3). Athletes from the amateur and professional levels were most often tested for aerobic capacity. These studies focused almost exclusively on male players (n = 9).
3.3.3. Acceleration-speed components
There are 66 articles in the present review, corresponding to six tests to assess on-ice acceleration and speed components in ice hockey. Forward acceleration or sprint are the most commonly used protocols (respectively n = 55; n = 45), followed by backward acceleration, backward sprint and full speed. As for skating distances, the most frequently applied protocols are the 6.1 m forward skating sprint test for on-ice acceleration and the 30 m for on-ice forward speed. All these are applied progressively for age cohort groups, with a prevalence among older athletes (n = 34 for 20-year-old group; n = 18 for 12–15 year-old group and n = 2 for ≤12 year-old group). From a level of play perspective, more tests were conducted at the competitive amateur level (n = 33) than at the youth (n = 26) or professional (n = 13) levels. This athletic attribute was more often assessed in North America than in Europe (nAmerica = 36; nEurope = 29).
3.3.4. Agility and change of direction abilities
On-ice agility and change of direction tests appeared 47 times in scientific publications. A high variability is observed in the selection of this category of tests, since 23 tests were found to evaluate this athletic quality, 17 of which appeared less than three times. Furthermore, there is a considerable gap in the literature regarding validation of agility tests, since only one test, the specific overall skating performance test (SOSPT), was validated over the timeframe of this review. The use of agility testing across the different studies was mostly targeted to male athletes (n = 42) aged 20 years and older (n = 23) playing at a competitive amateur level (n = 20). Only two studies were found regarding the assessment of agility in young ice hockey players under 12 years old. This on-ice attribute is assessed as much in North America as in Europe (nAmerica = 24; nEurope = 22), while Asia has one study. On an individual basis analysis, the Cornering S turn is the most frequently used on-ice test (n = 12) and implemented in both sexes. This protocol is well documented in the literature across the age ranges of 12–15, 16–19 and 20+ years old (n = 2; 4; 6) and is similarly represented in all levels of play (nyouth = 6; namateur = 4; npro = 3).
3.3.5. Ability to repeat skating sprints (RSSA)
Ability to repeat on-ice skating accelerations, sprints or intense effort tests are well documented in ice hockey assessment, with 41 articles corresponding to 19 different on-ice protocols. A substantial and similar variability in agility is also observed in the selection of this category of tests, since 13 of the 19 tests occurred less than three times. In the various studies, tests of skating sprint repetition ability involved mainly male athletes (n = 34) in an older population (i.e., 16–19 and over 20 years old; cumulated n = 44) compared to younger cohorts (under 12–15 and under 12 years old; cumulated n = 5) playing at a competitive amateur level (n = 22). From an individual perspective, the Reed test appears to be the most conducted test (n = 6), while others (i.e., repeated shift test, modified repeated sprint skating, endurance test, multiple repeated skate test, line drill) follow below (from n = 5 to n = 3).
3.4. Summary of on-ice protocols trends
Table 4 highlights observations and practical applications of usage trends for each physical quality assessed. SMAT is the most conducted test for on-ice aerobic capacity assessment and is both valid (r = 0.97) and reliable (r = 0.92) (105). Acceleration and speed variables are mainly assessed with 6.1 m forward skating and 30 m skating sprints with a high reliability (ICC ≥ 0.83, TE ≤ 0.5%) (63). The cornering S test is the most common protocol for evaluating on-ice agility and change of direction. Nevertheless, although there is no consensus on ability to repeat sprints, trends seem to establish that the Reed repeat sprint skate test and the repeated shift test are practical options for assessing this athletic component.
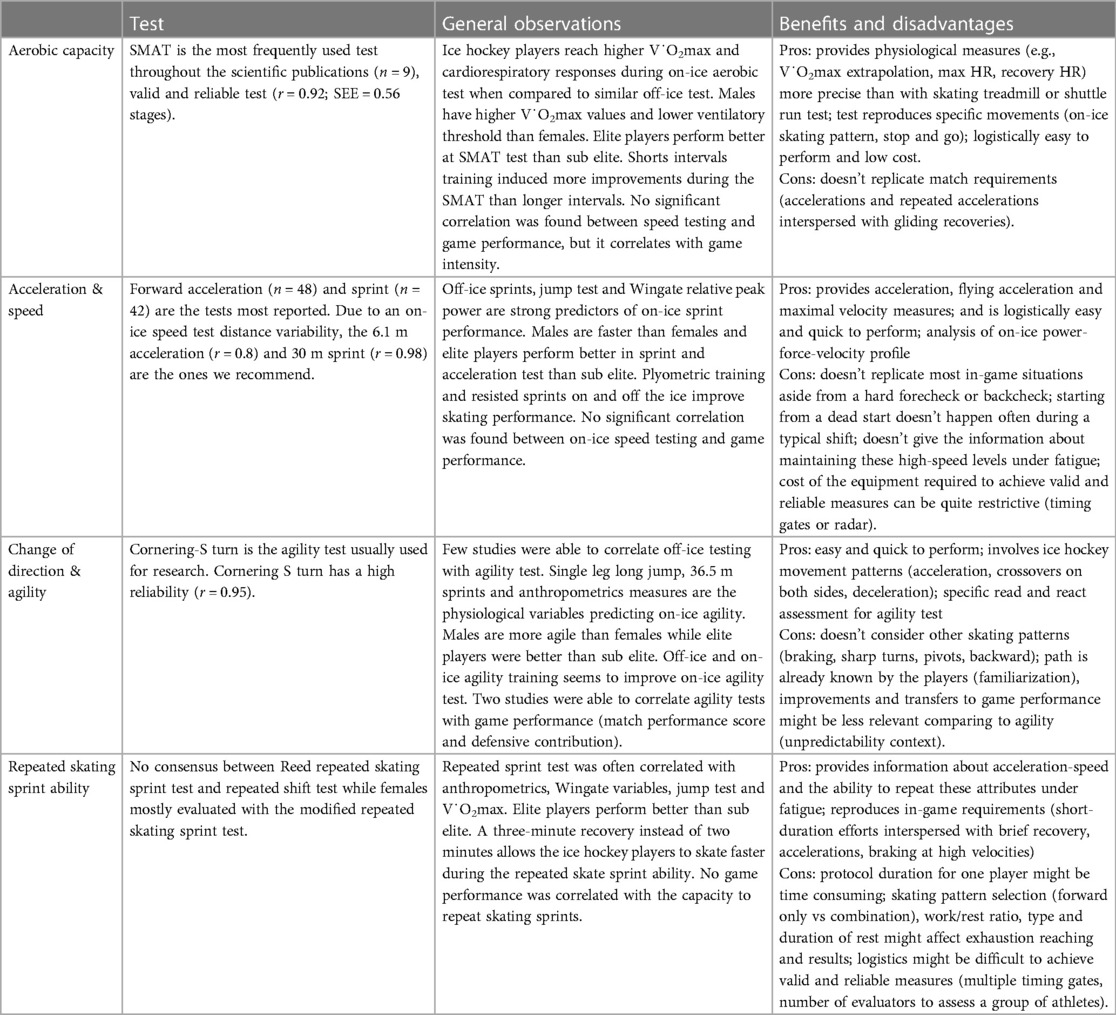
Table 4. Observation trends, benefits and constraints of the most used on-ice protocols.
4. Discussion
The general aim of this systematic review was to describe the extent to which evaluation protocols for different populations of ice hockey players have been used over the last twenty years. To this end, we identified four key categories of attributes related to ice hockey performance: aerobic capacity, speed, agility and ability to repeat intense efforts or sprints. Despite researchers’ efforts so far to document the usefulness of assessing hockey players (22), this review provides a complete overview of the work carried out in the specific fields of on-ice testing in ice hockey. Because on-ice tests are specifically linked to the actions of hockey players, we believe that stakeholders (researchers, strength coaches, coaching staff, etc.) can benefit from such an inventory by relying on tests adapted to the populations of athletes with whom they work. Considering the evolution of this sport and the physical characteristics of top-level hockey players (45, 82, 146), we limited our search to work published over the last two decades. We also excluded test protocols designed to measure technical or tactical skills, since this category refers mainly to young developing athletes. In this respect, hockey federations in most countries have already developed a list of tests based on their strategic orientations in terms of sports development (54, 147).
Regarding the populations studied and their attributes, it is interesting to note that the inventory of on-ice tests offers observations consistent with the attributes observed, as illustrated in Table 1. First, the on-ice agility and change of direction component predominates in the studies conducted with the youngest populations (e.g., 12–15 years old). This result is logical, given this attribute is a key element for young players aiming to further develop their hockey expertise; it is, moreover, consistent with most models of sports development (148). Our results suggest that young athletes under 12 years of age are relatively rarely evaluated, which is logically linked to the long-term athlete developmental stages. Such is not the case for their counterparts aged 16 and over, where evaluation becomes predominant in the progressive development of ice hockey-specific expertise (149). In more advanced populations (e.g., age group, level of play), we observe the importance of measuring the ability to repeat sprints, which is in line with the relevance of this on-ice performance indicator at the highest levels of competition (50, 92). Nevertheless, the most recent studies increasingly emphasize anaerobic (or hybrid) processes and their potential impact on performance in a game or competition context (145, 150, 151). In line with such results, the anaerobic component remains an important part of identifying potential NHL players, as it was demonstrated by Heller and colleagues (152) who tested elite Czech players. Our results for the acceleration and speed component, with a major utilization of 6.1 m and 30 m skating distances, are consistent with those of a previous systematic review (41).
As for test reliability and validity, results reveal that most of the protocols move in two opposite directions. First, the inventory proposed by our review suggests that most of the tests have very satisfactory levels of reliability and validity, at least with the populations studied using these protocols. Examples include the SMAT, 30-15 IIT, Yo-Yo IR, forward skating acceleration and sprint, cornering-S turn agility test, or even some ice hockey-specific repeated sprint tests such as the 7 × 15 m or the SOSPT. Another considerable part of these tests, however, has not been validated or replicated through an objective scientific process. This is mainly the case for tests targeting agility or ability to repeat intense skating efforts. Given their relevance, particularly for assessing the development of young talent, it is vital to identify and develop specific standardized, reliable and valid on-ice protocols to maintain qualitative analysis and optimal procedures to track progress and enable comparisons. Despite the lack of scientific insight, there is a definite advantage to assess in a sport-specific context that resembles a real game as closely as possible if the test is designed consistent with sport requirements (11, 104). As shown in Table 3, it exists a variability depending on the attribute evaluated. Aerobic and acceleration-speed capacities are assessed with a small range of tests (respectively n = 7 and 6), with a majority of them that have been validated and used in most research, in a logical manner. Conversely, a large variability has been highlighted in CoD-agility and ability to repeat skating sprints attributes, with a wide range of tests (respectively n = 19 and 23) developed to assess theses capacities in ice hockey athletes. Assess capacities as CoD, agility and RSSA in a specific ecological perspective on the ice has some issues in contrast of aerobic or speed qualities, which seems to be easier to standardize.
Most of the tests considered in this systematic review do not include the puck in the assessment, as described in Table 2. We think that it is logical mainly for practical reasons because puck loss during on-ice testing would lead to the athlete being required to repeat the test, which would increase the total evaluation time. Pucks could also damage specific measurement systems such as photoelectric cells. Therefore, it would be necessary to implement these on-ice tests both with and without pucks, to assess potential puck control differences and provide better support for each athlete. However, the use of the puck in on-ice acceleration, speed, CoD and agility tests could reveal an interesting detail about the offensive aspect of players. For example, a player who can reach a high percentage of his maximum on-ice skating speed while controlling the puck, as compared to a team-mate who degrades his skating speed in same conditions, is also an essential factor to consider for training and performance purposes.
This review also reveals that female players has so far received very little attention in the field of on-ice testing compared to their male counterparts. Considering the different physical capacities and the different role they can play in the selection process for female athletes, a more specific focus on the development of tests specific to female hockey players could offer some interesting advancements (11). In other words, it may not be optimal to consider the skills of female hockey players similarly to those of males given the game is structured differently, which could lend greater importance to other aspects.
Regarding level of play, our results indicate that most studies focus on the amateur sphere, with less attention paid to professional and youth levels. Data sensitivity at elite levels limits the possibility of evaluating athletes for research purpose, information is confidential and restricted to staff members who cannot communicate results because of privacy data protection. At youth levels, however, a plausible explanation could be that the development of technical on-ice skills takes priority over evaluation when an athlete lacks the necessary technical prerequisites, making evaluation needless. Our analysis reveals that most studies of on-ice performance evaluation are descriptive. This is understandable considering it’s easier to observe trends, relationships and correlations than to conduct more in-depth studies within structures, clubs and federations (e.g., longitudinal follow-up, effect of specific training on on-ice performance). Moreover, on-ice evaluation batteries are intended for formative or selective purposes.
4.1. Practical applications
The inventory proposed by this review helps identify the avenues to explore for a more enlightened view of the (evolving) status of ice hockey players worldwide. For researchers, it is a relevant guide that allows studies to be replicated based on the characteristics or variables being studied. An inventory of on-ice tests also gives researchers an indication of the populations under study. The tool enables teams’ coaches to make informed choices that will facilitate analysis and interpretation of the standards achieved by the populations under study. Coaches can also use on-ice testing by integrating it as a drill during practices to develop each attribute in a high pace manner. For example, agility test ended by a shot can be used at the start of practice to develop skills and agility to mimic game specific situations. Another option might be using RSSA test at the end of the practice and include several shots during each recovery time to develop the ability to perform and maintain precision shots despite fatigue and enjoying more the conditioning aspect of the drill. For strength and conditioning coaches, the assessment of all four areas of physical attributes is relevant for designing adapted training programs and verifying the outcomes of specific ice hockey conditioning training. It could also help them to better identify and select the off-ice tests which are closest to the on-ice tests most frequently used in the literature, with the aim of providing assessments that are more tailored to athletes’ needs.
4.2. Limitations and perspectives for future research
Despite the insights offered in this review, its limitations suggest avenues for future research. A first limitation is the identification of studies considering players’ on-ice fitness-performance. Here we considered only those published in academic journals. Some interesting approaches might reside in other types of publication, such as unpublished theses, hockey federations’ technical manuals or reports, and unpublished work. As an example, Hockey Canada has designed on-ice protocols and standards with a battery of tests, some based on the scientific literature and others that were empirically developed (54). Further research should also consider the on-ice tests designed and used mainly by federations and ice hockey clubs to analyze in depth the benefits of such assessments. However, the purpose of this study was to identify tests that were used and replicated in different research contexts. Additionally, we focused on a date range of the last twenty years of research (i.e., 2000–2002), a limitation in that we may have missed scientific articles published before that time, affecting proportions and results. Another limitation relates to emerging testing approaches that require advanced technologies such as global and local positioning systems (e.g., GPS, LPS), inertial movement units and object tracking methods. Since the reliability and validity of such technologies is well supported (16, 153), these promising approaches are now well established in soccer, while they have become more popular in the field of ice hockey research in recent years (36, 37, 154). From this perspective, we believe that such new and precise assessment methods will provide additional opportunities for researchers and professionals interested in measuring players’ attributes without testing them in the traditional ways identified in this review. This study finds that fewer ice hockey tests are conducted in female cohorts and that this trend should be reversed in future research so as to develop this research area and its practical application to female ice hockey athletes. Due to the large variability of testing procedures concerning agility and RSSA capacities, future research should also aim to determine which assessment is most highly related to in-game performance, despite the issues mentioned above, to validate and standardize an on-ice RSSA test useful for teams’ coaches and researchers.
5. Conclusion
Considering ice hockey match requirements and protocols used in the literature, the systematic review highlights the widespread use of on-ice skating tests to assess ability to repeat intense efforts, agility, acceleration and speed on the ice. Since on-ice tests relate specifically to the actions of the ice hockey player, we believe that ice hockey stakeholders can benefit from this practical and useful inventory tool through the guidance of tests adapted to the characteristics of the athlete populations they work with. Despite the issues and constraints of athletes’ testing, there is a need to assess as close as possible to real game conditions, on the ice with a full protective equipment, by using various specific skating patterns. This review proposes relevant options and solutions for researchers and practitioners (i.e., ice hockey coaches, on-ice skills specialists, strength and conditioning coaches, athletic therapists) who aim to integrate on-ice testing with different populations and objectives. The increasing emphasis on age-related on-ice evaluation indicates that ice hockey is a late-developing sport, where assessment becomes more relevant at an advanced age of expertise. Most research designs do not yet consider the associations between on-ice testing and performance in real competition settings. Indeed, live-match data are also complicated to collect, whether from an ethical, methodological or technological perspective. This review also suggests a need and relevance for developing and validating tests that assess ice hockey-specific skills and decision-making abilities. Performance in ice hockey is multi-factorial and depends on physical attributes (e.g., acceleration capacity, speed, power) and individual technical abilities (e.g., skating technique, capacity to change direction efficiently and quickly, passing accuracy). However, the interaction between the expression of these components and the unpredictable context of intermittent team sports remains an area that has not yet been fully assessed and decrypted. Agility is a complex but fundamental quality to evolve at the highest levels, refers to information and decision-making, playing intelligence, the core elements which each athlete must develop during their sporting career and which are challenging to evaluate from an ecological and specific perspective.
Data availability statement
The raw data supporting the conclusions of this article will be made available by the authors, without undue reservation.
Author contributions
JL led the systematic review project, MB and GM contributed equally and shared first authorship. MB, GM, FT, and JL contributed to the research conceptions and study design. MB and GM executed the study, investigated formal analysis and collected resources data. MB analyzed data, designed and organized the results tables and did the literature review process. GM and JL wrote the first draft of the article. All authors contributed to the article and approved the submitted version.
Funding
JL received a governmental grant from Ministère de l’Éducation du Québec [Initiative Projets Synergiques‘]. The project was also funded by MITACS (IT31707) between 2021 and 2023.
Acknowledgments
Authors acknowledge the contribution of UQTR Ice Hockey Research Laboratory group members for their help and participation in this research.
Conflict of interest
The authors declare that the research was conducted in the absence of any commercial or financial relationships that could be construed as a potential conflict of interest.
Publisher’s note
All claims expressed in this article are solely those of the authors and do not necessarily represent those of their affiliated organizations, or those of the publisher, the editors and the reviewers. Any product that may be evaluated in this article, or claim that may be made by its manufacturer, is not guaranteed or endorsed by the publisher.
References
1. Brocherie F, Girard O, Millet GP. Updated analysis of changes in locomotor activities across periods in an international ice hockey game. Biol Sport. (2018) 35(3):261–7. doi: 10.5114/biolsport.2018.77826
PubMed Abstract | CrossRef Full Text | Google Scholar
2. Neeld K. Preparing for the demands of professional hockey. Strength Cond J. (2018) 40(2):1–16. doi: 10.1519/ssc.0000000000000374
CrossRef Full Text | Google Scholar
5. Bracko MR, Fellingham GW. Comparison of physical performance characteristics of female and male ice hockey players. Pediatr Exerc Sci. (2001) 13(1):26–34. doi: 10.1123/pes.13.1.26
CrossRef Full Text | Google Scholar
6. Tarter BC, Kirisci L, Tarter RE, Weatherbee S, Jamnik V, McGuire EJ, et al. Use of aggregate fitness indicators to predict transition into the national hockey league. J Strength Cond Res. (2009) 23(6):1828–32. doi: 10.1519/JSC.0b013e3181b4372b
PubMed Abstract | CrossRef Full Text | Google Scholar
7. Gannon EA, Higham DG, Gardner BW, Nan N, Zhao J, Bisson LJ. Changes in neuromuscular status across a season of professional men’s ice hockey. J Strength Cond Res. (2021) 35(5):1338–44. doi: 10.1519/JSC.0000000000004001
PubMed Abstract | CrossRef Full Text | Google Scholar
8. Nightingale SC. A strength and conditioning approach for ice hockey. Strength Cond J. (2014) 36(6):28–36. doi: 10.1519/ssc.0000000000000107
CrossRef Full Text | Google Scholar
9. Daehlin TE, Haugen OC, Haugerud S, Hollan I, Raastad T, Ronnestad BR. Improvement of ice hockey players’ on-ice sprint with combined plyometric and strength training. Int J Sports Physiol Perform. (2017) 12(7):893–900. doi: 10.1123/ijspp.2016-0262
PubMed Abstract | CrossRef Full Text | Google Scholar
10. Lagrange S, Ferland PM, Leone M, Comtois AS. Contrast training generates post-activation potentiation and improves repeated sprint ability in elite ice hockey players. Int J Exerc Sci. (2020) 13(6):183–96.32148640
PubMed Abstract | Google Scholar
11. Lemoyne J, Brunelle JF, Huard Pelletier V, Glaude-Roy J, Martini G. Talent identification in elite adolescent ice hockey players: the discriminant capacity of fitness tests, skating performance and psychological characteristics. Sports. (2022) 10(4):58. doi: 10.3390/sports10040058
PubMed Abstract | CrossRef Full Text | Google Scholar
12. Vigh-Larsen JF, Beck JH, Daasbjerg A, Knudsen CB, Kvorning T, Overgaard K, et al. Fitness characteristics of elite and subelite male ice hockey players: a cross-sectional study. J Strength Cond Res. (2019) 33(9):2352–60. doi: 10.1519/JSC.0000000000003285
PubMed Abstract | CrossRef Full Text | Google Scholar
13. Martini G, Brunelle JF, Trudeau F, Lemoyne J. Measuring ice hockey skills in a repeated measures testing context: the effects of fatigue on skating efficiency, passing, agility, and shooting. Sport J. (2018) 21:1–16.
Google Scholar
14. Perez J, Guilhem G, Brocherie F. Reliability of the force-velocity-power variables during ice hockey sprint acceleration. Sports Biomech. (2022b) 21(1):56–70. doi: 10.1080/14763141.2019.1648541
PubMed Abstract | CrossRef Full Text | Google Scholar
15. Douglas AS, Rotondi MA, Baker J, Jamnik VK, Macpherson AK. A comparison of on-ice external load measures between subelite and elite female ice hockey players. J Strength Cond Res. (2022) 36(7):1978–83. doi: 10.1519/JSC.0000000000003771
PubMed Abstract | CrossRef Full Text | Google Scholar
16. Gamble ASD, Bigg JL, Pignanelli C, Nyman DLE, Burr JF, Spriet LL. Reliability and validity of an indoor local positioning system for measuring external load in ice hockey players. Eur J Sport Sci. (2023) 23(3):311–8. doi: 10.1080/17461391.2022.2032371
PubMed Abstract | CrossRef Full Text | Google Scholar
17. Cordingley DM, Sirant L, MacDonald PB, Leiter JR. Three-year longitudinal fitness tracking in top-level competitive youth ice hockey players. J Strength Cond Res. (2019) 33(11):2909–12. doi: 10.1519/JSC.0000000000003379
PubMed Abstract | CrossRef Full Text | Google Scholar
18. Martini G, Brunelle JF, Lalande V, Lemoyne J. Elite adolescent ice hockey players: analyzing associations between anthropometry, fitness, and on-ice performance. Int J Environ Res Public Health. (2022) 19(15):8952. doi: 10.3390/ijerph19158952
PubMed Abstract | CrossRef Full Text | Google Scholar
19. Worner T, Thorborg K, Eek F. Five-second squeeze testing in 333 professional and semiprofessional male ice hockey players: how are hip and groin symptoms, strength, and sporting function related? Orthop J Sports Med. (2019) 7(2):2325967119825858. doi: 10.1177/2325967119825858
PubMed Abstract | CrossRef Full Text | Google Scholar
20. Oliveras R, Bizzini M, Brunner R, Maffiuletti NA. Field-based evaluation of hip adductor and abductor strength in professional male ice hockey players: reference values and influencing factors. Phys Ther Sport. (2020) 43:204–9. doi: 10.1016/j.ptsp.2020.03.006
PubMed Abstract | CrossRef Full Text | Google Scholar
21. Delisle-Houde P, Reid RER, Insogna JA, Chiarlitti NA, Andersen RE. Seasonal changes in physiological responses and body composition during a competitive season in male and female elite collegiate ice hockey players. J Strength Cond Res. (2019b) 33(8):2162–9. doi: 10.1519/JSC.0000000000002338
PubMed Abstract | CrossRef Full Text | Google Scholar
22. Nightingale SC, Miller S, Turner A. The usefulness and reliability of fitness testing protocols for ice hockey players: a literature review. J Strength Cond Res. (2013) 27(6):1742–8. doi: 10.1519/JSC.0b013e3182736948
PubMed Abstract | CrossRef Full Text | Google Scholar
23. Cohen JN, Thompson KMA, Jamnik VK, Gledhill N, Burr JF. Relationship of fitness combine results and national hockey league performance: a 25-year analysis. Int J Sports Physiol Perform. (2022) 17(6):908–16. doi: 10.1123/ijspp.2021-0317
PubMed Abstract | CrossRef Full Text | Google Scholar
24. Vescovi JD, Murray TM, Fiala KA, VanHeest JL. Off-ice performance and draft status of elite ice hockey players. Int J Sports Physiol Perform. (2006) 1(3):207–21. doi: 10.1123/ijspp.1.3.207
PubMed Abstract | CrossRef Full Text | Google Scholar
25. Ferguson RJ, Marcotte G, Montpetit RR. A maximal oxygen uptake test during ice skating. Med Sci Sports. (1969) 1:207–11. doi: 10.1249/00005768-196912000-00007
CrossRef Full Text | Google Scholar
26. Hermiston RT, Gratto J, Teno T. Three hockey skills tests as predictors of hockey playing ability. Can J Appl Sport Sci. (1979) 4(1):95–7.498410
PubMed Abstract | Google Scholar
27. Reed A, Hasen H, Cotton C, Gauthier R, Jette M, Thoden J, et al. Development and validation of an on-ice hockey fitness test. Can J Appl Sport Sci. (1979) 4:245.
Google Scholar
28. Bracko MR. On-ice performance characteristics of elite and non-elite women’s ice hockey players. J Strength Cond Res. (2001) 15(1):42–7.11708705
PubMed Abstract | Google Scholar
29. Conners RT, Whitehead PN, Dodds FT, Schott KD, Quick MC. Validation of the polar team pro system for sprint speed with ice hockey players. J Strength Cond Res. (2022) 36(12):3468–72. doi: 10.1519/JSC.0000000000003784
PubMed Abstract | CrossRef Full Text | Google Scholar
30. Thompson KMA, Safadie A, Ford J, Burr JF. Off-ice resisted sprints best predict all-out skating performance in varsity hockey players. J Strength Cond Res. (2022) 36(9):2597–601. doi: 10.1519/JSC.0000000000003861
PubMed Abstract | CrossRef Full Text | Google Scholar
31. Bracko MR. Biomechanics powers ice hockey performance. Biomechanics. (2004) 9:47–53.
Google Scholar
32. Budarick AR, Shell JR, Robbins SMK, Wu T, Renaud PJ, Pearsall DJ. Ice hockey skating sprints: run to glide mechanics of high caliber male and female athletes. Sports Biomech. (2020) 19(5):601–17. doi: 10.1080/14763141.2018.1503323
PubMed Abstract | CrossRef Full Text | Google Scholar
33. Robbins SM, Renaud PJ, Pearsall DJ. Principal component analysis identifies differences in ice hockey skating stride between high- and low-calibre players. Sports Biomech. (2021) 20(2):131–49. doi: 10.1080/14763141.2018.1524510
PubMed Abstract | CrossRef Full Text | Google Scholar
34. Bracko MR, Fellingham GW, Hall LT, Fisher AG, Cryer W. Performance skating characteristics of professional ice hockey forwards. Sports Med Train Rehabil. (1998) 8(3):251–63. doi: 10.1080/15438629809512531
CrossRef Full Text | Google Scholar
35. Nadeau L, Godbout P, Richard J-F. Assessment of ice hockey performance in real-game conditions. Eur J Sport Sci. (2008) 8(6):379–88. doi: 10.1080/17461390802284456
CrossRef Full Text | Google Scholar
36. Van Iterson EH, Fitzgerald JS, Dietz CC, Snyder EM, Peterson BJ. Reliability of triaxial accelerometry for measuring load in men’s collegiate ice hockey. J Strength Cond Res. (2017) 31(5):1305–12. doi: 10.1519/JSC.0000000000001611
PubMed Abstract | CrossRef Full Text | Google Scholar
37. Douglas AS, Kennedy CR. Tracking in-match movement demands using local positioning system in world-class men’s ice hockey. J Strength Cond Res. (2020) 34(3):639–46. doi: 10.1519/JSC.0000000000003414
PubMed Abstract | CrossRef Full Text | Google Scholar
38. Perez J, Brocherie F, Couturier A, Guilhem G. International matches elicit stable mechanical workload in high-level female ice hockey. Biol Sport. (2022a) 39(4):857–64. doi: 10.5114/biolsport.2022.109455
PubMed Abstract | CrossRef Full Text | Google Scholar
39. Peterson BJ, Fitzgerald JS, Dietz CC, Ziegler KS, Baker SE, Snyder EM. Off-ice anaerobic power does not predict on-ice repeated shift performance in hockey. J Strength Cond Res. (2016) 30(9):2375–81. doi: 10.1519/JSC.0000000000001341
PubMed Abstract | CrossRef Full Text | Google Scholar
40. Chiarlitti NA, Crozier M, Insogna JA, Reid RER, Delisle-Houde P. Longitudinal physiological and fitness evaluations in elite ice hockey: a systematic review. J Strength Cond Res. (2021) 35(10):2963–79. doi: 10.1519/JSC.0000000000004115
PubMed Abstract | CrossRef Full Text | Google Scholar
41. Stastny P, Musalek M, Roczniok R, Cleather D, Novak D, Vagner M. Testing distance characteristics and reference values for ice-hockey straight sprint speed and acceleration. A systematic review and meta-analyses. Biol Sport. (2023) 40:899–918. doi: 10.5114/biolsport.2023.122479
PubMed Abstract | CrossRef Full Text | Google Scholar
42. Huard Pelletier V, Glaude-Roy J, Daigle AP, Brunelle JF, Bissonnette A, Lemoyne J. Associations between testing and game performance in ice hockey: a scoping review. Sports. (2021) 9(9):117. doi: 10.3390/sports9090117
PubMed Abstract | CrossRef Full Text | Google Scholar
43. Baumeister RF. Writing a literature review. In: Prinstein MJ, editors. The portable mentor. New York, NY, US: Springer Science Business Media (2013). p. 119–32.
44. Page MJ, McKenzie JE, Bossuyt PM, Boutron I, Hoffmann TC, Mulrow CD, et al. The PRISMA 2020 statement: an updated guideline for reporting systematic reviews. Syst Rev. (2021) 10(1):89. doi: 10.1186/s13643-021-01626-4
PubMed Abstract | CrossRef Full Text | Google Scholar
45. Triplett AN, Ebbing AC, Green MR, Connolly CP, Carrier DP, Pivarnik JM. Changes in collegiate ice hockey player anthropometrics and aerobic fitness over 3 decades. Appl Physiol Nutr Metab. (2018) 43(9):950–5. doi: 10.1139/apnm-2017-0789
PubMed Abstract | CrossRef Full Text | Google Scholar
47. Methley AM, Campbell S, Chew-Graham C, McNally R, Cheraghi-Sohi S. PICO, PICOS and SPIDER: a comparison study of specificity and sensitivity in three search tools for qualitative systematic reviews. BMC Health Serv Res. (2014) 14:579. doi: 10.1186/s12913-014-0579-0
PubMed Abstract | CrossRef Full Text | Google Scholar
48. Vigh-Larsen JF, Mohr M. The physiology of ice hockey performance: an update. Scand J Med Sci Sports. (2022) 00:1–14. doi: 10.1111/sms.14284
CrossRef Full Text | Google Scholar
49. Nightingale SC. Ice hockey: the validity and reliability of a novel on-ice test for ice hockey players. Professional Strength Cond. (2013) 31:15–8.
Google Scholar
50. Novak D, Lipinska P, Roczniok R, Spieszny M, Stastny P. Off-ice agility provide motor transfer to on-ice skating performance and agility in adolescent ice hockey players. J Sports Sci Med. (2019) 18(4):680–94.31827353
PubMed Abstract | Google Scholar
51. Girard O, Mendez-Villanueva A, Bishop D. Repeated-sprint ability – part I: factors contributing to fatigue. Sports Med. (2011) 41(8):673–94. doi: 10.2165/11590550-000000000-00000
PubMed Abstract | CrossRef Full Text | Google Scholar
52. Stanula A, Gupta S, Baron J, Bieniec A, Tomik R, Gabrys T, et al. A comparative study of two-minute versus three-minute passive recovery on sprint skating performance of ice hockey forwards and defensemen. Int J Environ Res Public Health. (2021) 18(24):10591. doi: 10.3390/ijerph182413029
PubMed Abstract | CrossRef Full Text | Google Scholar
53. International Ice Hockey Federation. “Youth Olympic Games National Skills Challenge Operations Manual” (2020).
55. Balyi I. Long-term Planning of Athlete Development, Multiple Periodization, Modeling and Normative Data (1999).
56. Allisse M, Bui HT, Desjardins P, Léger L, Comtois A-S, Leone M. Assessment of on-ice oxygen cost of skating performance in elite youth ice hockey players. J Strength Cond Res. (2021) 35(12):3466–73. doi: 10.1519/JSC.0000000000003324
PubMed Abstract | CrossRef Full Text | Google Scholar
57. Allisse M, Bui HT, Léger L, Comtois A-S, Leone M. Updating the skating multistage aerobic test and correction for V˙O2max prediction using a new skating economy index in elite youth ice hockey players. J Strength Cond Res. (2020) 34(11):3182–9. doi: 10.1519/jsc.0000000000002602
PubMed Abstract | CrossRef Full Text | Google Scholar
58. Allisse M, Sercia P, Comtois A-S, Leone M. Morphological, physiological and skating performance profiles of male age-group elite ice hockey players. J Hum Kinet. (2017) 58(1):87–97. doi: 10.1515/hukin-2017-0085
PubMed Abstract | CrossRef Full Text | Google Scholar
59. Baron J, Gupta S, Bieniec A, Klich G, Gabrys T, Swinarew AS, et al. Effect of rest period duration between sets of repeated sprint skating ability test on the skating ability of ice hockey players. Int J Environ Res Public Health. (2021) 18(20):10591. doi: 10.3390/ijerph182010591
PubMed Abstract | CrossRef Full Text | Google Scholar
60. Behm DG, Wahl MJ, Button DC, Power KE, Anderson KG. Relationship between hockey skating speed and selected performance measures. J Strength Cond Res. (2005) 19(2):326–31. doi: 10.1519/R-14043.1
PubMed Abstract | CrossRef Full Text | Google Scholar
61. Besson C, Buchheit M, Praz M, Dériaz O, Millet GP. Cardiorespiratory responses to the 30-15 intermittent ice test. Int J Sports Physiol Perform. (2013) 8(2):173–80. doi: 10.1123/ijspp.8.2.173
PubMed Abstract | CrossRef Full Text | Google Scholar
62. Boland M, Delude K, Miele EM. Relationship between physiological off-ice testing, on-ice skating, and game performance in division I female ice hockey players. J Strength Cond Res. (2019) 33(6):1619–28. doi: 10.1519/JSC.0000000000002265
PubMed Abstract | CrossRef Full Text | Google Scholar
63. Bond CW, Bennett TW, Noonan BC. Evaluation of skating top speed, acceleration, and multiple repeated sprint speed ice hockey performance tests. J Strength Cond Res. (2018) 32(8):2273–83. doi: 10.1519/JSC.0000000000002644
PubMed Abstract | CrossRef Full Text | Google Scholar
64. Boucher VG, Parent A-A, Miron FS-J, Leone M, Comtois AS. Comparison between power off-ice test and performance on-ice anaerobic testing. J Strength Cond Res. (2020) 34(12):3498–505. doi: 10.1519/JSC.0000000000002336
PubMed Abstract | CrossRef Full Text | Google Scholar
65. Bracko MR, George JD. Prediction of ice skating performance with off-ice testing in women’s ice hockey players. J Strength Cond Res. (2001) 15(1):116–22.11708693
PubMed Abstract | Google Scholar
66. Brocherie F, Perez J, Guilhem G. Effects of a 14-day high-intensity shock microcycle in high-level ice hockey players’ fitness. J Strength Cond Res. (2022) 36(8):2247–52. doi: 10.1519/JSC.0000000000003769
PubMed Abstract | CrossRef Full Text | Google Scholar
67. Buchheit M, Lefebvre B, Laursen PB, Ahmaidi S. Reliability, usefulness, and validity of the 30-15 intermittent ice test in young elite ice hockey players. J Strength Cond Res. (2011) 25(5):1457–64. doi: 10.1519/JSC.0b013e3181d686b7
PubMed Abstract | CrossRef Full Text | Google Scholar
68. Buckeridge E, LeVangie MC, Stetter B, Nigg SR, Nigg BM. An on-ice measurement approach to analyse the biomechanics of ice hockey skating. PLoS One. (2015) 10(5):e0127324. doi: 10.1371/journal.pone.0127324
PubMed Abstract | CrossRef Full Text | Google Scholar
69. Byrkjedal PT, Bjørnsen T, Luteberget LS, Lindberg K, Ivarsson A, Haukali E, et al. Association between physical performance tests and external load during scrimmages in highly trained youth ice hockey players. Int J Sports Physiol Perform. (2022) 18(1):47–54. doi: 10.1123/ijspp.2022-0225
PubMed Abstract | CrossRef Full Text | Google Scholar
70. Carey DG, Drake MM, Pliego GJ, Raymond RL. Do hockey players need aerobic fitness? Relation between V˙O2max and fatigue during high-intensity intermittent ice skating. J Strength Cond Res. (2007) 21(3):963–6. doi: 10.1519/R-18881.1
PubMed Abstract | CrossRef Full Text | Google Scholar
71. Czeck MA, Roelofs EJ, Dietz C, Bosch TA, Dengel DR. Body composition and on-ice skate times for national collegiate athletic association division I collegiate male and female ice hockey athletes. J Strength Cond Res. (2022) 36(1):187–92. doi: 10.1519/JSC.0000000000004175
PubMed Abstract | CrossRef Full Text | Google Scholar
72. Daigle A-P, Bélanger S, Brunelle J-F, Lemoyne J. Functional performance tests, on-ice testing and game performance in elite junior ice hockey players. J Hum Kinet. (2022) 83(1):245–56. doi: 10.2478/hukin-2022-000076
PubMed Abstract | CrossRef Full Text | Google Scholar
73. Delisle-Houde P, Chiarlitti NA, Reid RE, Andersen RE. Predicting on-ice skating using laboratory-and field-based assessments in college ice hockey players. Int J Sports Physiol Perform. (2019a) 14(9):1184–9. doi: 10.1123/ijspp.2018-0708
CrossRef Full Text | Google Scholar
74. Durocher JJ, Guisfredi AJ, Leetun DT, Carter JR. Comparison of on-ice and off-ice graded exercise testing in collegiate hockey players. Appl Physiol Nutr Metab. (2010) 35(1):35–9. doi: 10.1139/H09-129
PubMed Abstract | CrossRef Full Text | Google Scholar
75. Durocher JJ, Jensen DD, Arredondo AG, Leetun DT, Carter JR. Gender differences in hockey players during on-ice graded exercise. J Strength Cond Res. (2008a) 22(4):1327–31. doi: 10.1519/JSC.0b013e31816eb4c1
PubMed Abstract | CrossRef Full Text | Google Scholar
76. Durocher JJ, Leetun DT, Carter JR. Sport-specific assessment of lactate threshold and aerobic capacity throughout a collegiate hockey season. Appl Physiol Nutr Metab. (2008b) 33(6):1165–71. doi: 10.1139/H08-107
PubMed Abstract | CrossRef Full Text | Google Scholar
77. Eliason PH, McKay CD, Meeuwisse WH, Hagel BE, Nadeau L, Emery CA. History of previous concussion and sports-specific skills in youth ice hockey players. J Phys Educ Sport. (2020) 20(3):2174–81. doi: 10.7752/jpes.2020.s3292
CrossRef Full Text | Google Scholar
78. Farlinger CM, Fowles JR. The effect of sequence of skating-specific training on skating performance. Int J Sports Physiol Perform. (2008) 3(2):185–98. doi: 10.1123/ijspp.3.2.185
PubMed Abstract | CrossRef Full Text | Google Scholar
79. Farlinger CM, Kruisselbrink LD, Fowles JR. Relationships to skating performance in competitive hockey players. J Strength Cond Res. (2007) 21(3):915–22. doi: 10.1519/R-19155.1
PubMed Abstract | CrossRef Full Text | Google Scholar
80. Federolf P, Redmond A. Does skate sharpening affect individual skating performance in an agility course in ice hockey? Sports Eng. (2010) 13:39–46. doi: 10.1007/s12283-010-0050-3
CrossRef Full Text | Google Scholar
81. Federolf P, Nigg B. Skating performance in ice hockey when using a flared skate blade design. Cold Reg Sci Technol. (2012) 70:12–8. doi: 10.1016/j.coldregions.2011.08.009
CrossRef Full Text | Google Scholar
82. Ferland PM, Marcotte-L’Heureux V, Roy P, Carey VD, Charron J, Lagrange S, et al. Maximal oxygen consumption requirements in professional North American ice hockey. J Strength Cond Res. (2021) 35(6):1586–92. doi: 10.1519/JSC.0000000000003966
PubMed Abstract | CrossRef Full Text | Google Scholar
83. Geithner CA. Predicting performance in women’s ice hockey. In: Duncan M, Lyons M, editors. Advances in strength and conditioning research. New York, NY: Nova Science Publishers (2009). p. 51–63.
84. Geithner CA, Lee AM, Bracko MR. Physical and performance differences among forwards, defensemen, and goalies in elite women’s ice hockey. J Strength Cond Res. (2006) 20(3):500–5. doi: 10.1519/17375.1
PubMed Abstract | CrossRef Full Text | Google Scholar
85. Gilenstam KM, Thorsen K, Henriksson-Larsén KB. Physiological correlates of skating performance in women’s and men’s ice hockey. J Strength Cond Res. (2011) 25(8):2133–42. doi: 10.1519/JSC.0b013e3181ecd072
PubMed Abstract | CrossRef Full Text | Google Scholar
86. Girdauskas G, Kazakevičius R. Optimization of technical training of ice-hockey players aged 8–17 years. Ugdymas. Kūno kultūra. Sportas. (2013) 2:19–26. doi: 10.33607/bjshs.v2i89.155
CrossRef Full Text | Google Scholar
87. Gupta S, Baron J, Bieniec A, Swinarew A, Stanula A. Relationship between vertical jump tests and ice-skating performance in junior Polish ice hockey players. Biol Sport. (2022) 40(1):225–32. doi: 10.5114/biolsport.2023.112972
PubMed Abstract | CrossRef Full Text | Google Scholar
88. Hajek F, Keller M, Taube W, von Duvillard SP, Bell JW, Wagner H. Testing-specific skating performance in ice hockey. J Strength Cond Res. (2021) 35:S70–5. doi: 10.1519/JSC.0000000000003475
PubMed Abstract | CrossRef Full Text | Google Scholar
89. Haukali E, Tjelta LI. Correlation between “off-ice” variables and skating performance among young male ice hockey players. Int J Appl Sports Sci. (2015) 27(1):26–32. doi: 10.24985/ijass.2015.27.1.26
CrossRef Full Text | Google Scholar
90. Haukali E, Tjelta LI. Relationship between off-season changes in power and in-season changes in skating speed in young ice hockey players. Int J Appl Sports Sci. (2016) 28(2):111–22. doi: 10.24985/ijass.2016.28.2.111
CrossRef Full Text | Google Scholar
91. Henriksson T, Vescovi JD, Fjellman-Wiklund A, Gilenstam K. Laboratory-and field-based testing as predictors of skating performance in competitive-level female ice hockey. Open Access J Sports Med. (2016) 7:81. doi: 10.2147/OAJSM.S109124
PubMed Abstract | CrossRef Full Text | Google Scholar
92. Hůlka K, Bělka J, Cuberek R, Schneider O. Reliability of specific on-ice repeated-sprint ability test for ice-hockey players. Acta Univ Palacki Olomuc Gymn. (2014) 44(2):69–75. doi: 10.5507/ag.2014.007
CrossRef Full Text | Google Scholar
93. Jackson J, Snydmiller G, Game A, Gervais P, Bell G. Investigation of positional differences in fitness of male university ice hockey players and the frequency, time spent and heart rate of movement patterns during competition. Int J Kinesiol Sports Sci. (2017) 5(3):6–15. doi: 10.7575/aiac.ijkss.v.5n.3p.6
CrossRef Full Text | Google Scholar
94. Janot JM, Auner KA, Emberts TM, Kaatz RM, Matteson KM, Muller EA, et al. The effects of bungeeskate training on measures of on-ice acceleration and speed. Int J Sports Physiol Perform. (2013) 8(4):419–27. doi: 10.1123/ijspp.8.4.419
PubMed Abstract | CrossRef Full Text | Google Scholar
95. Janot JM, Beltz NM, Dalleck LD. Multiple off-ice performance variables predict on-ice skating performance in male and female division III ice hockey players. J Sports Sci Med. (2015) 14(3):522–9.26336338
PubMed Abstract | Google Scholar
96. Kaartinen S, Venojärvi M, Lesch KJ, Tikkanen H, Vartiainen P, Stenroth L. Lower limb muscle activation patterns in ice-hockey skating and associations with skating speed. Sports Biomech. (2021):1–16. doi: 10.1080/14763141.2021.2014551 [Epub ahead of print].34930101
PubMed Abstract | CrossRef Full Text | Google Scholar
97. Kinnunen J-V, Piitulainen H, Piirainen JM. Neuromuscular adaptations to short-term high-intensity interval training in female ice-hockey players. J Strength Cond Res. (2019) 33(2):479–85. doi: 10.1519/JSC.0000000000001881
PubMed Abstract | CrossRef Full Text | Google Scholar
98. Knechta M, Čillík I, Zháněl J. Influence of plyometric training on the level of speed ability with changes of direction in ice hockey. Stud Sport. (2021) 15(1):17–25. doi: 10.5817/StS2021-1-2
CrossRef Full Text | Google Scholar
99. Knechta M. Impact of explosive strength of lower limbs on skating and running speed on a 10 m distance in 14–15 years old ice hockey players: a recent study. Humanit Soc Sci. (2021) 1:30–7. doi: 10.9734/bpi/sthss/v1/9029D
CrossRef Full Text | Google Scholar
100. Krause DA, Smith AM, Holmes LC, Klebe CR, Lee JB, Lundquist KM, et al. Relationship of off-ice and on-ice performance measures in high school male hockey players. J Strength Cond Res. (2012) 26(5):1423–30. doi: 10.1519/JSC.0b013e318251072d
PubMed Abstract | CrossRef Full Text | Google Scholar
101. Lamoureux NR, Tomkinson GR, Peterson BJ, Fitzgerald JS. Relationship between skating economy and performance during a repeated-shift test in elite and subelite ice hockey players. J Strength Cond Res. (2018) 32(4):1109–13. doi: 10.1519/JSC.0000000000002418
PubMed Abstract | CrossRef Full Text | Google Scholar
102. Lau S, Berg K, Latin RW, Noble J. Comparison of active and passive recovery of blood lactate and subsequent performance of repeated work bouts in ice hockey players. J Strength Cond Res. (2001) 15(3):367–71. doi: 10.1519/1533-4287(2001)015%3C0367:COAAPR%3E2.0.CO;2
PubMed Abstract | CrossRef Full Text | Google Scholar
104. Legerlotz K, Kittelmann J, Dietzel M, Wolfarth B, Bohlke N. Ice hockey-specific repeated shuttle sprint test performed on ice should not be replaced by off-ice testing. J Strength Cond Res. (2022) 36(4):1071–6. doi: 10.1519/JSC.0000000000003576
PubMed Abstract | CrossRef Full Text | Google Scholar
105. Leone M, Léger LA, Larivière G, Comtois AS. An on-ice aerobic maximal multistage shuttle skate test for elite adolescent hockey players. Int J Sports Med. (2007) 28(10):823–8. doi: 10.1055/s-2007-964986
PubMed Abstract | CrossRef Full Text | Google Scholar
106. Lignell E, Fransson D, Krustrup P, Mohr M. Analysis of high-intensity skating in top-class ice hockey match-play in relation to training status and muscle damage. J Strength Cond Res. (2018) 32(5):1303–10. doi: 10.1519/JSC.0000000000001999
PubMed Abstract | CrossRef Full Text | Google Scholar
107. Lowery MR, Tomkinson GR, Peterson BJ, Fitzgerald JS. The relationship between ventilatory threshold and repeated-sprint ability in competitive male ice hockey players. J Exerc Sci Fit. (2018) 16(1):32–6. doi: 10.1016/j.jesf.2018.03.003
PubMed Abstract | CrossRef Full Text | Google Scholar
108. Madden RF, Erdman KA, Shearer J, Spriet LL, Ferber R, Kolstad AT, et al. Effects of caffeine on exertion, skill performance, and physicality in ice hockey. Int J Sports Physiol Perform. (2019) 14(10):1422–9. doi: 10.1123/ijspp.2019-0130
PubMed Abstract | CrossRef Full Text | Google Scholar
109. Matthews MJ, Comfort P, Crebin R. Complex training in ice hockey: the effects of a heavy resisted sprint on subsequent ice-hockey sprint performance. J Strength Cond Res. (2010) 24(11):2883–7. doi: 10.1519/JSC.0b013e3181e7253c
PubMed Abstract | CrossRef Full Text | Google Scholar
110. Naimo M, De Souza E, Wilson J, Carpenter A, Gilchrist P, Lowery R, et al. High-intensity interval training has positive effects on performance in ice hockey players. Int J Sports Med. (2015) 36(01):61–6. doi: 10.1055/s-0034-1382054
PubMed Abstract | CrossRef Full Text | Google Scholar
111. Nigg CR, Gessner A, Nigg C, Giurgiu M, Neumann R. Demographische, physiologische, psychologische und on-ice leistungsindikatoren sagen die plus/minus-statistik von freizeit-eishockeyspielern über eine saison voraus. Ger J Exerc Sport Res. (2020) 50:463–9. doi: 10.1007/s12662-020-00679-2
CrossRef Full Text | Google Scholar
112. Nobes K, Montgomery D, Pearsall D, Turcotte R, Lefebvre R, Whittom F. A comparison of skating economy on-ice and on the skating treadmill. Can J Appl Physiol. (2003) 28(1):1–11. doi: 10.1139/h03-001
PubMed Abstract | CrossRef Full Text | Google Scholar
113. Novak D, Tomasek A, Lipinska P, Stastny P. The specificity of motor learning tasks determines the kind of skating skill development in older school-age children. Sports. (2020) 8(9):126. doi: 10.3390/sports8090126
PubMed Abstract | CrossRef Full Text | Google Scholar
114. Opáth L. Powerskating as a method of skating development in category older students and youth team. J Phys Educ Sport Health. (2015) 4(2):17–21.
Google Scholar
115. Paľov R. Influence of the time of the day and chronotype on speed abilities in junior team ice hockey players. SportLogia. (2014) 10(2):122–8. doi: 10.5550/sgia.141002.en.009P
CrossRef Full Text | Google Scholar
116. Perez J, Guilhem G, Brocherie F. Ice hockey forward skating force-velocity profiling using single unloaded vs. multiple loaded methods. J Strength Cond Res. (2021a) 36(11):3229–33. doi: 10.1519/jsc.0000000000004078
PubMed Abstract | CrossRef Full Text | Google Scholar
117. Perez J, Guilhem G, Hager R, Brocherie F. Mechanical determinants of forward skating sprint inferred from off-and on-ice force-velocity evaluations in elite female ice hockey players. Eur J Sport Sci. (2021b) 21(2):192–203. doi: 10.1080/17461391.2020.1751304
PubMed Abstract | CrossRef Full Text | Google Scholar
118. Peterson BJ, Fitzgerald JS, Dietz CC, Ziegler KS, Ingraham SJ, Baker SE, et al. Division I hockey players generate more power than division III players during on-and off-ice performance tests. J Strength Cond Res. (2015b) 29(5):1191–6. doi: 10.1519/JSC.0000000000000754
PubMed Abstract | CrossRef Full Text | Google Scholar
119. Peterson BJ, Fitzgerald JS, Dietz CC, Ziegler KS, Ingraham SJ, Baker SE, et al. Aerobic capacity is associated with improved repeated shift performance in hockey. J Strength Cond Res. (2015a) 29(6):1465–72. doi: 10.1519/JSC.0000000000000786
PubMed Abstract | CrossRef Full Text | Google Scholar
120. Petrella NJ, Montelpare WJ, Nystrom M, Plyley M, Faught BE. Validation of the FAST skating protocol to predict aerobic power in ice hockey players. Appl Physiol Nutr Metab. (2007) 32(4):693–700. doi: 10.1139/H07-057
PubMed Abstract | CrossRef Full Text | Google Scholar
121. Peyer KL, Pivarnik JM, Eisenmann JC, Vorkapich M. Physiological characteristics of national collegiate athletic association division I ice hockey players and their relation to game performance. J Strength Cond Res. (2011) 25(5):1183–92. doi: 10.1519/JSC.0b013e318217650a
PubMed Abstract | CrossRef Full Text | Google Scholar
122. Potteiger JA, Smith DL, Maier ML, Foster TS. Relationship between body composition, leg strength, anaerobic power, and on-ice skating performance in division I men’s hockey athletes. J Strength Cond Res. (2010) 24(7):1755–62. doi: 10.1519/JSC.0b013e3181e06cfb
PubMed Abstract | CrossRef Full Text | Google Scholar
123. Power A, Faught B, Przysucha E, McPherson M, Montelpare W. Establishing the test–retest reliability and concurrent validity for the repeat ice skating test (RIST) in adolescent male ice hockey players. Meas Phys Educ Exerc Sci. (2012) 16(1):69–80. doi: 10.1080/1091367X.2012.639618
CrossRef Full Text | Google Scholar
124. Rago V, Muschinsky A, Deylami K, Vigh-Larsen JF, Mohr M. Game demands of a professional ice hockey team with special emphasis on fatigue development and playing position. J Hum Kinet. (2022) 84:195–205. doi: 10.2478/hukin-2022-000078
PubMed Abstract | CrossRef Full Text | Google Scholar
125. Roczniok R, Maszczyk A, Czuba M, Stanula A, Pietraszewski P, Gabryś T. The predictive value of on-ice special tests in relation to various indexes of aerobic and anaerobic capacity in ice hockey players. Hum Mov. (2012) 13(1):28–32. doi: 10.2478/v10038-012-0001-x
CrossRef Full Text | Google Scholar
126. Roczniok R, Maszczyk A, Stanula A, Czuba M, Pietraszewski P, Kantyka J, et al. Physiological and physical profiles and on-ice performance approach to predict talent in male youth ice hockey players during draft to hockey team. Isokinet Exerc Sci. (2013) 21(2):121–7. doi: 10.3233/ies-130487
CrossRef Full Text | Google Scholar
127. Roczniok R, Stanula A, Gabryś T, Szmatlan-Gabryś U, Gołaś A, Stastny P. Physical fitness and performance of polish ice-hockey players competing at different sports levels. J Hum Kinet. (2016a) 51(1):201–8. doi: 10.1515/hukin-2015-0165
PubMed Abstract | CrossRef Full Text | Google Scholar
128. Roczniok R, Stanula A, Maszczyk A, Mostowik A, Kowalczyk M, Fidos-Czuba O, et al. Physiological, physical and on-ice performance criteria for selection of elite ice hockey teams. Biol Sport. (2016b) 33(1):43–8. doi: 10.5604/20831862.1180175
PubMed Abstract | CrossRef Full Text | Google Scholar
129. Rønnestad BR, Haugen OC, Dæhlin TE. Superior on-ice performance after short-interval vs. long-interval training in well-trained adolescent ice hockey players. J Strength Cond Res. (2021) 35:S76–80. doi: 10.1519/jsc.0000000000004113
CrossRef Full Text | Google Scholar
130. Runner AR, Lehnhard RA, Butterfield SA, Tu S, O’Neill T. Predictors of speed using off-ice measures of college hockey players. J Strength Cond Res. (2016) 30(6):1626–32. doi: 10.1519/jsc.0000000000000911
PubMed Abstract | CrossRef Full Text | Google Scholar
131. Schwesig R, Hermassi S, Edelmann S, Thorhauer U, Schulze S, Fieseler G, et al. Relationship between ice hockey-specific complex test and maximal strength, aerobic capacity and postural regulation in professional players. J Sports Med Phys Fit. (2017) 57(11):1415–23. doi: 10.23736/s0022-4707.17.07020-7
CrossRef Full Text | Google Scholar
132. Secomb JL, Dascombe BJ, Nimphius S. Importance of joint angle-specific hip strength for skating performance in semiprofessional ice hockey athletes. J Strength Cond Res. (2021) 35(9):2599–603. doi: 10.1519/JSC.0000000000004087
PubMed Abstract | CrossRef Full Text | Google Scholar
133. Shell JR, Robbins SM, Dixon PC, Renaud PJ, Turcotte RA, Wu T, et al. Skating start propulsion: three-dimensional kinematic analysis of elite male and female ice hockey players. Sports Biomech. (2017) 16(3):313–24. doi: 10.1080/14763141.2017.1306095
PubMed Abstract | CrossRef Full Text | Google Scholar
134. Skowronek T, Socha T, Roczniok R, Socha S. The predictive value of various anaerobic capacity indices in relation to specific on-ice performance tests in ice hockey players. Life Sci J. (2013) 10(4):2228–832.
Google Scholar
135. Slavicek T, Stastny P, Roczniok R, Musalek M. Lower limb skeletal robustness determines the change of directional speed performance in youth ice hockey. J Hum Kinet. (2022) 85:75–85. doi: 10.2478/hukin-2022-0111
PubMed Abstract | CrossRef Full Text | Google Scholar
136. Smith AM, Krause DA, Stuart MJ, Montelpare WJ, Sorenson MC, Link AA, et al. Skating crossovers on a motorized flywheel: a preliminary experimental design to test effect on speed and on crossovers. J Strength Cond Res. (2013) 27(12):3412–8. doi: 10.1519/JSC.0b013e3182915f37
PubMed Abstract | CrossRef Full Text | Google Scholar
137. Stanula A, Roczniok R, Maszczyk A, Pietraszewski P, Zajac A. The role of aerobic capacity in high-intensity intermittent efforts in ice-hockey. Biol Sport. (2014) 31(3):193–9. doi: 10.5604/20831862.1111437
PubMed Abstract | CrossRef Full Text | Google Scholar
138. Steeves D, Campagna P. The relationship between maximal aerobic power and recovery in elite ice hockey players during a simulated game. J Strength Cond Res. (2019) 33(9):2503–12. doi: 10.1519/JSC.0000000000002506
PubMed Abstract | CrossRef Full Text | Google Scholar
139. Stenroth L, Vartiainen P, Karjalainen PA. Force-velocity profiling in ice hockey skating: reliability and validity of a simple, low-cost field method. Sports Biomech. (2020) 22(7):1–16. doi: 10.1080/14763141.2020.1770321
CrossRef Full Text | Google Scholar
140. Stetter BJ, Buckeridge E, Nigg SR, Sell S, Stein T. Towards a wearable monitoring tool for in-field ice hockey skating performance analysis. Eur J Sport Sci. (2019) 19(7):893–901. doi: 10.1080/17461391.2018.1563634
PubMed Abstract | CrossRef Full Text | Google Scholar
141. Vigh-Larsen JF, Ermidis G, Rago V, Randers MB, Fransson D, Nielsen JL, et al. Muscle metabolism and fatigue during simulated ice hockey match-play in elite players. Med Sci Sports Exercise. (2020a) 52(10):2162–71. doi: 10.1249/MSS.0000000000002370
CrossRef Full Text | Google Scholar
142. Vigh-Larsen JF, Haverinen MT, Panduro J, Ermidis G, Andersen TB, Overgaard K, et al. On-ice and off-ice fitness profiles of elite and U20 male ice hockey players of two different national standards. J Strength Cond Res. (2020b) 34(12):3369–76. doi: 10.1519/JSC.0000000000003836
PubMed Abstract | CrossRef Full Text | Google Scholar
143. Wagner H, Abplanalp M, von Duvillard SP, Bell JW, Taube W, Keller M. The relationship between on-ice and off-ice performance in elite male adolescent ice hockey players—an observation study. Appl Sci. (2021) 11(6):2724. doi: 10.3390/app11062724
CrossRef Full Text | Google Scholar
144. Williams M, Grau S. Physical performance and the relationship to game performance in elite adolescent ice hockey. Int J Strength Conf. (2020) 1(1):1–11. doi: 10.47206/iuscaj.v1i1.3
CrossRef Full Text | Google Scholar
145. Wilson K, Jackson J, Snydmiller G, Bell G. Development and reliability of a 7 (15 m repeated on-ice sprint test for female ice hockey players. Int J Exerc Sci. (2021) 14(6):666–76.34567374
PubMed Abstract | Google Scholar
146. Ransdell LB, Murray TM, Gao Y. Off-ice fitness of elite female ice hockey players by team success, age, and player position. J Strength Cond Res. (2013) 27(4):875–84. doi: 10.1519/JSC.0b013e3182651fd2
PubMed Abstract | CrossRef Full Text | Google Scholar
147. Hockey Canada. “Plan de Hockey Canada pour le développement à long terme du joueur”.) (2013).
148. Higgs C, Way R, Harber V, Jurbala P, Balyi I. “Développement à long terme par le sport et l’activité physique”, C.S. Institute, 3rd edition (2019).
149. Lloyd RS, Cronin JB, Faigenbaum AD, Haff GG, Howard R, Kraemer WJ, et al. National strength and conditioning association position statement on long-term athletic development. J Strength Cond Res. (2016) 30(6):1491–509. doi: 10.1519/JSC.0000000000001387
PubMed Abstract | CrossRef Full Text | Google Scholar
150. Laakso L. Critical evaluation of the physiological adaptations to repeated-sprint training: implications for training recommendations and repeated-sprint ability of well-trained field-based team-sport athletes. J Aust Strength Cond Res. (2020) 28(4):75–81.
Google Scholar
151. Schwesig R, Laudner KG, Delank K-S, Brill R, Schulze S. Relationship between ice hockey-specific complex test (IHCT) and match performance. Appl Sci. (2021) 11(7):3080. doi: 10.3390/app11073080
CrossRef Full Text | Google Scholar
152. Heller J, Vodicka P, Janek M. Anaerobic performance in 30s wingate test as one of the possible criteria for selection Czech hockey players into national hockey league. Phys Act Rev. (2019) 7:57–62. doi: 10.16926/par.2019.07.07
CrossRef Full Text | Google Scholar
153. Luteberget LS, Gilgien M. Validation methods for global and local positioning-based athlete monitoring systems in team sports: a scoping review. BMJ Open Sport Exerc Med. (2020) 6(1):e000794. doi: 10.1136/bmjsem-2020-000794
PubMed Abstract | CrossRef Full Text | Google Scholar
154. Vats K, Walters P, Fani M, Clausi DA, Zelek JS. Player tracking and identification in ice hockey. Expert Syst Appl. (2023) 213:119250. doi: 10.1016/j.eswa.2022.119250
CrossRef Full Text | Google Scholar